Traditionally, organisms are divided into heterotrophs and autotrophs, depending on their source of energy and building blocks. Autotrophs get the necessary nutrients from simple inorganic molecules – prime example is photosynthetic organisms – while heterotrophs need to consume organic compounds full of energy, such as sugars and lipids. This difference determines which organism can live where and by extension the food relationships in the natural environment. Plants and algae are normally autotrophic, while animals and fungi are heterotrophic. Bacteria can be either, but a species needs to specialize in a particular mode of feeding. The characterization of an organism as a heterotroph or an autotroph is so fundamental that switching between categories would be considered unthinkable up to a few years ago. But this is no longer the case.
As the (plant-derived) sugars could be better used to feed the population, and as the need to reduce the atmospheric CO2 levels becomes more and more imminent, scientists started wondering if it would be possible to rewire the microbes’ metabolism to use carbon dioxide instead. In principle, this should be possible. Nature found ways to use CO2 a billion years ago, and synthetic biology can move metabolic pathways from one organism to the other. The next logical step is therefore to transfer this carbon fixation ability to model bacteria and yeasts.
The most common carbon assimilation metabolic pathway is the Calvin-Benson-Bassam cycle, where carbon dioxide, with the help of the enzyme RUBISCO gets incorporated into an organic molecule (a sugar). This sugar provides the chemical building blocks and fuels the cell’s metabolism. In theory, if we express the Calvin cycle enzymes into heterotrophic bacteria or yeasts they should be able to perform carbon fixation. This was shown in 2016, when Israeli researchers, led by Professor Ron Milo, enabled a bacterium to form some of its biomass out of carbon dioxide. But making the same microbe generate all its biomass out of atmospheric gasses is far more complicated.
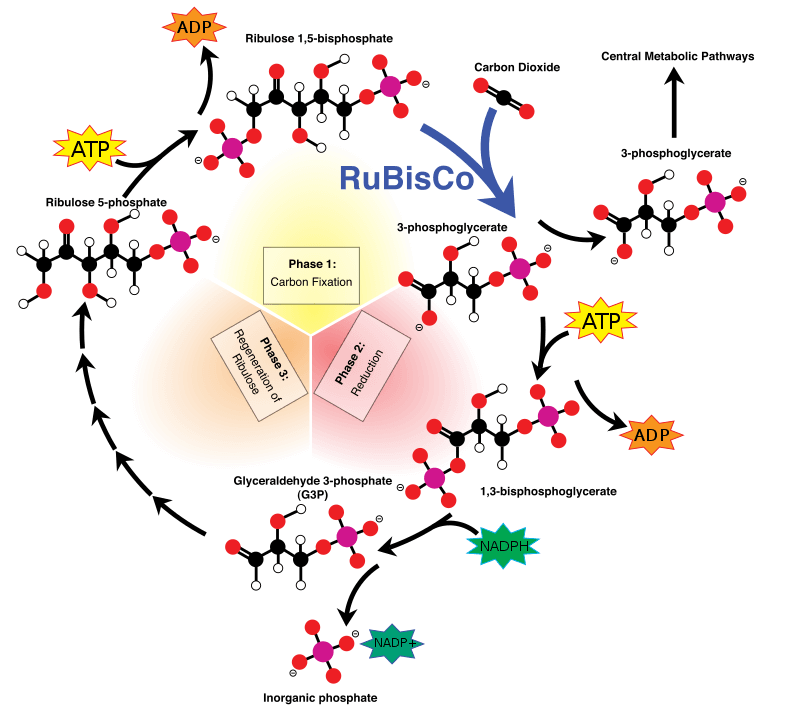
Escherichia coli and Pichia pastoris are two microbes commonly used in biotechnology. Both are easy to grow. Both are easy to genetically engineer. Both grow optimally using sugars as a feedstock. And as of last year, both can grow autotrophically using only CO2 as their carbon source. Milo’s research group continued their work in E. coli, and reported in Cell the generation of a strain that can only grow in high concentrations of CO2. At the same time, researchers from Austria generated a P. pastoris strain that does the same, as reported in Nature Biotechnology. Even though the two organisms are very different, the two research groups used similar strategies to achieve autotrophy. First, they introduced the foreign enzymes that enable carbon fixation. Then, they made the organism dependent on the new metabolic route by erasing enzymes involved in the sugar metabolism. Finally, they performed laboratory evolution which increased the carbon fixation rates and the growth in carbon dioxide. It is very interesting to note that the yeast strain was growing better when the carbon-fixing enzymes were expressed into peroxisomes – specialized organelles that host redox enzymes.
These two works are significant scientific landmarks. Bacteria and yeasts are adapted to consuming sugars, and the researchers managed to completely rewire their native metabolisms with a few targeted interventions and adaptation experiments. However, there are still challenges to overcome before making these strains biotechnologically relevant. The most important of them are the very slow growth rate and the requirement of high CO2 concentrations. But these two strains will be important tools to fundamentally understand autotrophy and large-scale “lifestyle” changes in microorganisms.
Biotechnology is nowadays taking a sustainability direction. Whether it comes in the form of employing microbes to sequester greenhouse gasses, or using photosynthetic microorganisms for biotech purposes, scientists and the industry are optimistic that biotechnology can give reliable solutions to the greenhouse gas problem. Is a carbon-fixing E. coli or P. pastoris part of this solution? Potentially, but not necessarily. A lot of the press coverage around these two research works hails them as a solution to the rising CO2 levels, where the engineered strains will drain the atmosphere of harmful gasses and turn them into useful chemicals. In reality, the industrial challenge doesn’t lie on performing carbon fixation (there are organisms that already do that, and they do it well) but on how to better tie up industrial practices. In essence, we need to assure that the waste of one process is directly used into another and not released in the environment.
In that sense, having a flexible organism, easily adapted to different environments, and ready to consume any compound would be a valuable tool. The lessons learned from this research though can guide major “lifestyle changes” to other industrial microbes to fill a specific need. Or they can be the basis for further strain improvements in E. coli and P. pastoris, making them grow on more greenhouse gasses or increase their carbon-fixation and growth rate. But we shouldn’t expect a slow-growing CO2-consuming microbe to turn the industry upside down on its own.
Kostas Vavitsas, PhD, is a Senior Research Associate at the University of Athens, Greece. He is also a steering committee member of EUSynBioS. Follow him on Twitter @konvavitsas